
Upon a sudden increase in temperature or other stresses (red lightning bolt), fewer proteins maintain their correct shape (rectangles) misfolded proteins (stars) therefore accumulate in the cell. These data are the strongest evidence to date that Hsp70 feedback regulates the heat shock response by directly associating with Hsf1, as happens in the chaperone titration model ( Figure 1).Ĭlockwise from top: The chaperone protein Hsp70 binds to the heat shock transcription factor Hsf1, repressing its transcriptional activity. Further support for the chaperone titration model came from experiments in which yeast cells expressed two types of Hsf1: wild-type Hsf1 and “decoy” Hsf1 (which can bind to Hsp70 but cannot activate the transcription of the Hsf1-dependent genes).
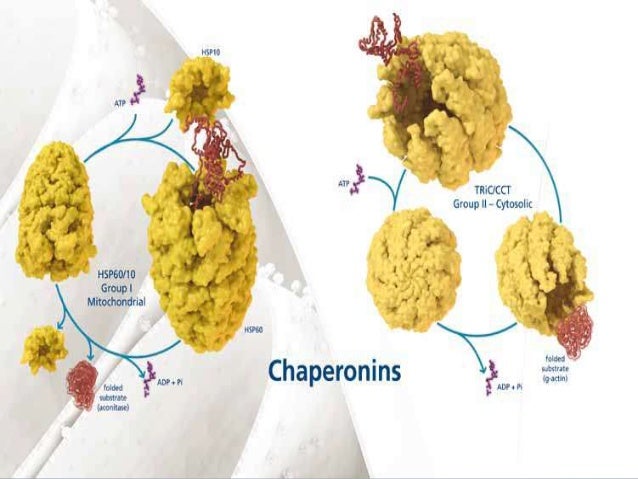
simulated how the expression of Hsf1-dependent genes changes in response to interactions between Hsp70, Hsf1 and misfolded proteins. Initial experimental results demonstrated that a chaperone called Hsp70 – which is thought to damp down the heat shock response – binds to Hsf1 under non-stress conditions and is released upon a sudden increase in temperature. Now, in eLife, David Pincus and coworkers from the Whitehead Institute for Biomedical Research, Boston University and Harvard University – including Xu Zheng and Joanna Krakowiak as joint first authors – report how they have used a combination of mathematical modeling and cell biology experiments in yeast to address these issues ( Zheng et al., 2016). In addition, Hsf1 is subject to a large number of post-translational modifications, such as phosphorylation, but the influence of these modifications on the heat shock response is a topic of controversy ( Budzyński et al., 2015 Xia and Voellmy, 1997). Although there is evidence to support such a model, it was unclear which – if any – of the chaperones is the main regulator of the Hsf1 activity cycle. The chaperone titration model assumes that Hsf1 is kept inactive in unstressed cells by its interactions with chaperones the presence of misfolded proteins then activates Hsf1 by attracting (or “titrating”) the chaperones away from Hsf1. This model can only apply to animal cells because Hsf1 forms trimers in yeast under all conditions, even in the absence of heat shock ( Sorger et al., 1987). The intrinsic response model, which assumes that Hsf1 directly senses increasing temperature (and potentially other stresses), relies on Hsf1 transitioning from a monomer to a trimer ( Zhong et al., 1998 Hentze et al., 2016). The heat shock response has been studied for more than 30 years, mainly in yeast and animal cells, and different models have been proposed to explain it ( Anckar and Sistonen, 2011).
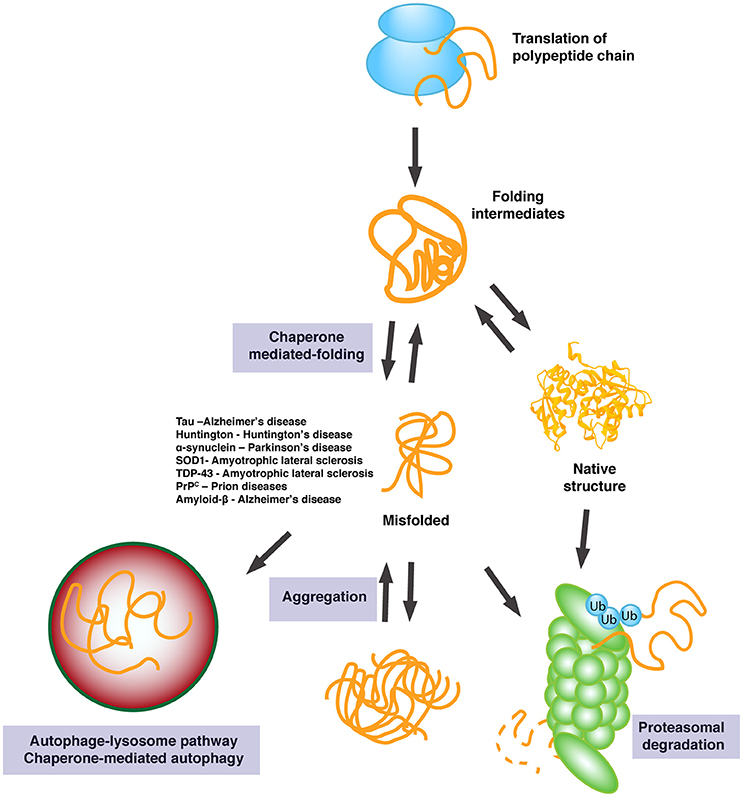
Once the effects of the stress have been dealt with, cells reduce the production of heat shock proteins to normal levels. These proteins include the chaperones that refold misfolded proteins or target them for degradation. Three copies of this protein combine to form trimers that bind to the promoters of Hsf1-dependent genes, which leads to higher levels of heat shock proteins inside the cell. This response is an evolutionarily conserved transcriptional program that is orchestrated in eukaryotic cells by a protein called Hsf1 (short for heat shock factor 1 Morimoto, 1998). Such stresses – which can be caused by a range of conditions, including changes in temperature and mechanical stresses – are damaging to proteins, so cells mount the so-called heat shock response. Cells are subjected to frequent assaults either from their environment or as a consequence of development or disease.
